Artificial Photosynthesis: Can We Harness the Energy of the Sun as Well as Plants?
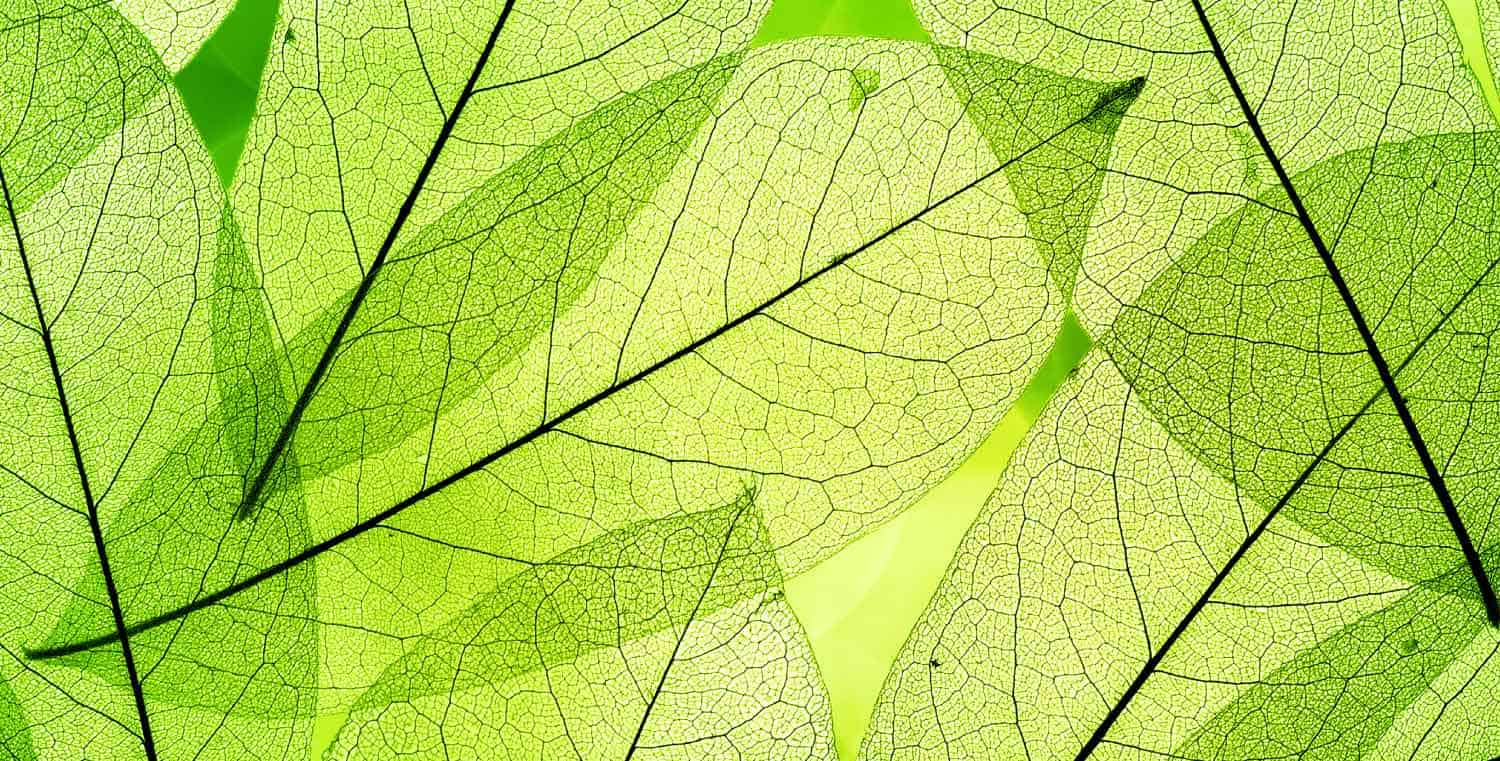
Contents
Click here to see this page in other languages : Russian
In the early 1900s, the Italian chemist Giacomo Ciamician recognized that fossil fuel use was unsustainable. And like many of today’s environmentalists, he turned to nature for clues on developing renewable energy solutions, studying the chemistry of plants and their use of solar energy. He admired their unparalleled mastery of photochemical synthesis—the way they use light to synthesize energy from the most fundamental of substances—and how “they reverse the ordinary process of combustion.”
In photosynthesis, Ciamician realized, lay an entirely renewable process of energy creation. When sunlight reaches the surface of a green leaf, it sets off a reaction inside the leaf. Chloroplasts, energized by the light, trigger the production of chemical products—essentially sugars—which store the energy such that the plant can later access it for its biological needs. It is an entirely renewable process; the plant harvests the immense and constant supply of solar energy, absorbs carbon dioxide and water, and releases oxygen. There is no other waste.
If scientists could learn to imitate photosynthesis by providing concentrated carbon dioxide and suitable catalyzers, they could create fuels from solar energy. Ciamician was taken by the seeming simplicity of this solution. Inspired by small successes in chemical manipulation of plants, he wondered, “does it not seem that, with well-adapted systems of cultivation and timely intervention, we may succeed in causing plants to produce, in quantities much larger than the normal ones, the substances which are useful to our modern life?”
In 1912, Ciamician sounded the alarm about the unsustainable use of fossil fuels, and he exhorted the scientific community to explore artificially recreating photosynthesis. But little was done. A century later, however, in the midst of a climate crisis, and armed with improved technology and growing scientific knowledge, his vision reached a major breakthrough.
After more than ten years of research and experimentation, Peidong Yang, a chemist at UC Berkeley, successfully created the first photosynthetic biohybrid system (PBS) in April 2015. This first-generation PBS uses semiconductors and live bacteria to do the photosynthetic work that real leaves do—absorb solar energy and create a chemical product using water and carbon dioxide, while releasing oxygen—but it creates liquid fuels. The process is called artificial photosynthesis, and if the technology continues to improve, it may become the future of energy.
How Does This System Work?
Yang’s PBS can be thought of as a synthetic leaf. It is a one-square-inch tray that contains silicon semiconductors and living bacteria; what Yang calls a semiconductor-bacteria interface.
In order to initiate the process of artificial photosynthesis, Yang dips the tray of materials into water, pumps carbon dioxide into the water, and shines a solar light on it. As the semiconductors harvest solar energy, they generate charges to carry out reactions within the solution. The bacteria take electrons from the semiconductors and use them to transform, or reduce, carbon dioxide molecules and create liquid fuels. In the meantime, water is oxidized on the surface of another semiconductor to release oxygen. After several hours or several days of this process, the chemists can collect the product.
With this first-generation system, Yang successfully produced butanol, acetate, polymers, and pharmaceutical precursors, fulfilling Ciamician’s once-far-fetched vision of imitating plants to create the fuels that we need. This PBS achieved a solar-to-chemical conversion efficiency of 0.38%, which is comparable to the conversion efficiency in a natural, green leaf.
Describing his research, Yang says, “Our system has the potential to fundamentally change the chemical and oil industry in that we can produce chemicals and fuels in a totally renewable way, rather than extracting them from deep below the ground.”
If Yang’s system can be successfully scaled up, businesses could build artificial forests that produce the fuel for our cars, planes, and power plants by following the same laws and processes that natural forests follow. Since artificial photosynthesis would absorb and reduce carbon dioxide in order to create fuels, we could continue to use liquid fuel without destroying the environment or warming the planet.
However, in order to ensure that artificial photosynthesis can reliably produce our fuels in the future, it has to be better than nature, as Ciamician foresaw. Our need for renewable energy is urgent, and Yang’s model must be able to provide energy on a global scale if it is eventually to replace fossil fuels.
Recent Developments in Yang’s Artificial Photosynthesis
Since the major breakthrough in April 2015, Yang has continued to improve his system in hopes of eventually producing fuels that are commercially viable, efficient, and durable.
In August 2015, Yang and his team tested his system with a different type of bacteria. The method is the same, except instead of electrons, the bacteria use molecular hydrogen from water molecules to reduce carbon dioxide and create methane, the primary component of natural gas. This process is projected to have an impressive conversion efficiency of 10%, which is much higher than the conversion efficiency in natural leaves.
A conversion efficiency of 10% could potentially be commercially viable, but since methane is a gas it is more difficult to use than liquid fuels such as butanol, which can be transferred through pipes. Overall, this new generation of PBS needs to be designed and assembled in order to achieve a solar-to-liquid-fuel efficiency above 10%.
In December 2015, Yang advanced his system further by making the remarkable discovery that certain bacteria could grow the semiconductors by themselves. This development short-circuited the two-step process of growing the nanowires and then culturing the bacteria in the nanowires. The improved semiconductor-bacteria interface could potentially be more efficient in producing acetate, as well as other chemicals and fuels, according to Yang. And in terms of scaling up, it has the greatest potential.
In the past few weeks, Yang made yet another important breakthrough in elucidating the electron transfer mechanism between the semiconductor-bacteria interface. This sort of fundamental understanding of the charge transfer at the interface will provide critical insights for the designing of the next generation PBS with better efficiency and durability. He will be releasing the details of this breakthrough shortly.
Despite these important breakthroughs and modifications to the PBS, Yang clarifies, “the physics of the semiconductor-bacteria interface for the solar driven carbon dioxide reduction is now established.” As long as he has an effective semiconductor that absorbs solar energy and feeds electrons to the bacteria, the photosynthetic function will initiate, and the remarkable process of artificial photosynthesis will continue to produce liquid fuels.
Why This Solar Power Is Unique
Peter Forbes, a science writer and the author of Nanoscience: Giants of the Infinitesimal, admires Yang’s work in creating this system. He writes, “It’s a brilliant synthesis: semiconductors are the most efficient light harvesters, and biological systems are the best scavengers of CO2.”
Yang’s artificial photosynthesis only relies on solar energy. But it creates a more useable source of energy than solar panels, which are currently the most popular and commercially viable form of solar power. While the semiconductors in solar panels absorb solar energy and convert it into electricity, in artificial photosynthesis, the semiconductors absorb solar energy and store it in “the carbon-carbon bond or the carbon-hydrogen bond of liquid fuels like methane or butanol.”
This difference is crucial. The electricity generated from solar panels simply cannot meet our diverse energy needs, but these renewable liquid fuels and natural gases can. Unlike solar panels, Yang’s PBS absorbs and breaks down carbon dioxide, releases oxygen, and creates a renewable fuel that can be collected and used. With artificial photosynthesis creating our fuels, driving cars and operating machinery becomes much less harmful. As Katherine Bourzac phrases nicely, “This is one of the best attempts yet to realize the simple equation: sun + water + carbon dioxide = sustainable fuel.”
The Future of Artificial Photosynthesis
Yang’s PBS has been advancing rapidly, but he still has work to do before the technology can be considered commercially viable. Despite encouraging conversion efficiencies, especially with methane, the PBS is not durable enough or cost-effective enough to be marketable.
In order to improve this system, Yang and his team are working to figure out how to replace bacteria with synthetic catalysts. So far, bacteria have proven to be the most efficient catalysts, and they also have high selectivity—that is, they can create a variety of useful compounds such as butanol, acetate, polymers and methane. But since bacteria live and die, they are less durable than a synthetic catalyst and less reliable if this technology is scaled up.
Yang has been testing PBS’s with live bacteria and synthetic catalysts in parallel systems in order to discover which type works best. “From the point of view of efficiency and selectivity of the final product, the bacteria approach is winning,” Yang says, “but if down the road we can find a synthetic catalyst that can produce methane and butanol with similar selectivity, then that is the ultimate solution.” Such a system would give us the ideal fuels and the most durable semiconductor-catalyst interface that can be reliably scaled up.
Another concern is that, unlike natural photosynthesis, artificial photosynthesis requires concentrated carbon dioxide to function. This is easy to do in the lab, but if artificial photosynthesis is scaled up, Yang will have to find a feasible way of supplying concentrated carbon dioxide to the PBS. Peter Forbes argues that Yang’s artificial photosynthesis could be “coupled with carbon-capture technology to pull CO2 from smokestack emissions and convert it into fuel”. If this could be done, artificial photosynthesis would contribute to a carbon-neutral future by consuming our carbon emissions and releasing oxygen. This is not the focus of Yang’s research, but it is an integral piece of the puzzle that other scientists must provide if artificial photosynthesis is to supply the fuels we need on a large scale.
When Giacomo Ciamician considered the future of artificial photosynthesis, he imagined a future of abundant energy where humans could master the “photochemical processes that hitherto have been the guarded secret of the plants…to make them bear even more abundant fruit than nature, for nature is not in a hurry and mankind is.” And while the rush was not apparent to scientists in 1912, it is clear now, in 2016.
Peidong Yang has already created a system of artificial photosynthesis that out-produces nature. If he continues to increase the efficiency and durability of his PBS, artificial photosynthesis could revolutionize our energy use and serve as a sustainable model for generations to come. As long as the sun shines, artificial photosynthesis can produce fuels and consume waste. And in this future of artificial photosynthesis, the world would be able to grow and use fuels freely; knowing that the same, natural process that created them would recycle the carbon at the other end.
Yang shares this hope for the future. He explains, “Our vision of a cyborgian evolution—biology augmented with inorganic materials—may bring the PBS concept to full fruition, selectively combining the best of both worlds, and providing society with a renewable solution to solve the energy problem and mitigate climate change.”
If you would like to learn more about Peidong Yang’s research, please visit his website at http://nanowires.berkeley.edu/.
About the Future of Life Institute
The Future of Life Institute (FLI) is a global think tank with a team of 20+ full-time staff operating across the US and Europe. FLI has been working to steer the development of transformative technologies towards benefitting life and away from extreme large-scale risks since its founding in 2014. Find out more about our mission or explore our work.
Related content
Other posts about Biotech, Climate & Environment, Recent News
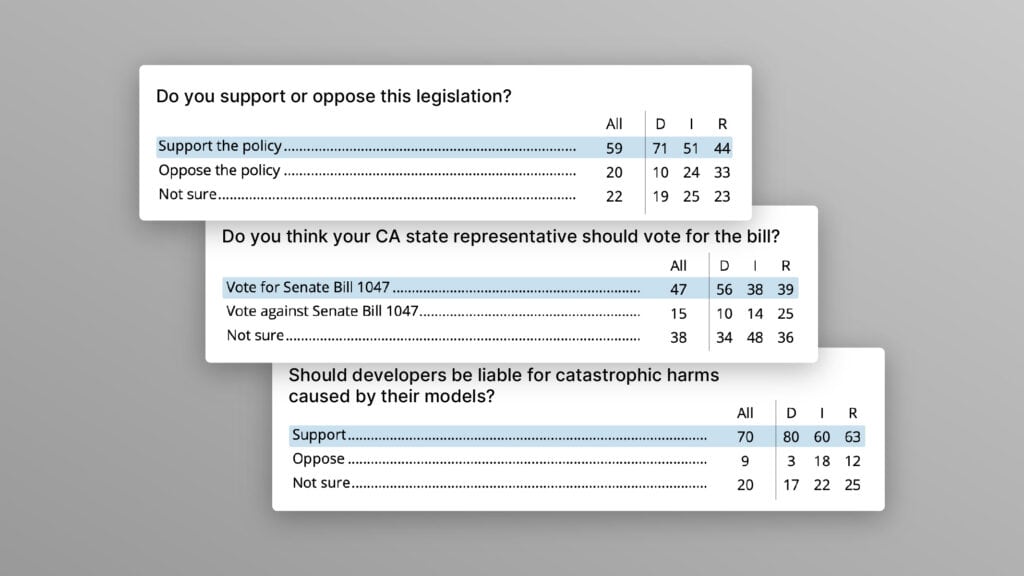
Poll Shows Broad Popularity of CA SB1047 to Regulate AI
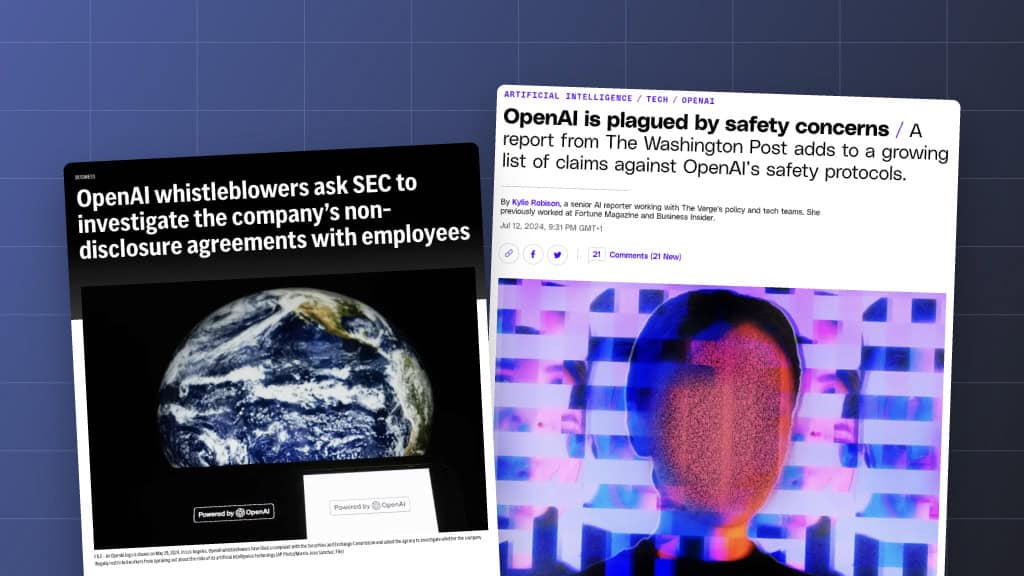